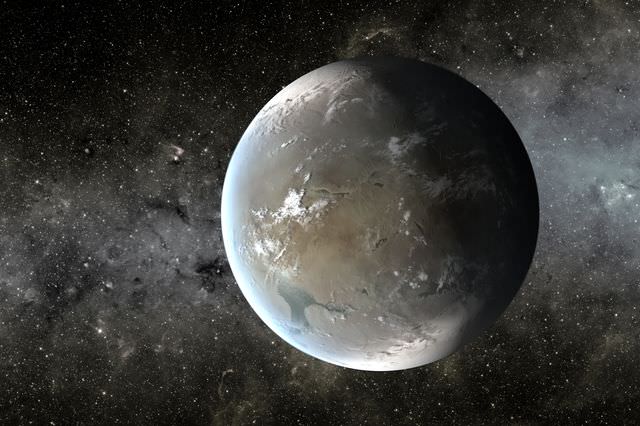
Less than a year after it went to space, the James Webb Space Telescope (JWST) has already demonstrated its worth many times over. The images it has acquired of distant galaxies, nebulae, exoplanet atmospheres, and deep fields are the most detailed and sensitive ever taken. And yet, one of the most exciting aspects of its mission is just getting started: the search for evidence of life beyond Earth. This will consist of Webb using its powerful infrared instruments to look for chemical signatures associated with life and biological processes (aka. biosignatures).
The chemical signatures vary, each representing a different pathway toward the potential discovery of life. According to The Conversation’s Joanna Barstow, a planetary scientist and an Ernest Rutherford Fellow at The Open University specializing in the study of exoplanet atmospheres, there are four ways that Webb could do this. These include looking for chemicals that lifeforms depend on, chemical byproducts produced by living organisms, chemicals essential to maintaining a stable climate, and chemicals that shouldn’t coexist.
In their search for life beyond Earth, astrobiologists have been restricted to the “low-hanging fruit” approach. This consists of searching for terrestrial (or rocky) planets that orbit within their parent stars’ circumsolar habitable zones (HZs) or the distance where planets will be warm enough to maintain liquid water on their surfaces. With next-generation telescopes like Webb, which combine sensitive optics, coronographs, and spectrometers with near- and mid-infrared imaging capability, the field of exoplanet study is transitioning from discovery to characterization.
As we explored in previous articles, this involves direct imaging studies of exoplanets and obtaining spectroscopic data from their atmospheres. Since the early 19th century, scientists have known that certain chemical elements absorb light at certain wavelengths and radiate it in others. By performing a “chemical inventory” of exoplanet atmospheres, astrobiologists will be able to place much tighter constraints on exoplanet habitability. In other words, they will be able to say with much greater confidence if a planet is habitable (and not just “potentially habitable”).
That being said, the chemical indicators that Webb could look for (as Barstow explains) can be broken down into four groups: Oxygen and Ozone, Phosphine and Ammonia, Methane and Carbon Dioxide, and Chemical Imbalances. Oxygen is an obvious biosignature because of its importance to the emergence and maintenance of life here on Earth. In Earth’s early history, our atmosphere was largely composed of carbon dioxide, and oxidization was prevented by removing oxygen (aka. a “reducing” atmosphere).
Over time, cyanobacteria and other photosynthetic organisms converted atmospheric CO2 into oxygen gas. This culminated in the “Great Oxygenation Event” (GOE) roughly 2.4 to 2.0 billion years ago, when Earth’s atmosphere went from a “reducing” to an “oxidizing” atmosphere. This allowed more complex organisms like insects, birds, mammals, and (eventually) hominids to evolve and flourish. Then there’s Ozone, which formed in Earth’s upper atmosphere from the interaction of oxygen gas and ultraviolet (UV) radiation.
This led to the creation of the Ozone Layer, which today protects life on Earth from the majority of the Sun’s UV radiation. However, finding these molecules in an exoplanet’s atmosphere does not necessarily mean we’ve found evidence of life. As multiple studies have shown, there are numerous ways that a planet’s atmosphere can become oxidizing through the creation of “abiotic oxygen” (aka. not the result of biological processes). As Barstow indicated, another scenario involves a “runaway greenhouse effect,” where evaporating surface water leads to more heating and evaporation in a feedback loop.
The presence of so much water vapor in the planet’s atmosphere will lead to photolysis, where exposure to solar radiation causes water to break down into hydrogen and oxygen gas (the former of which is lost to space while the latter is retained). In another scenario, tidally-locked planets that orbit within a star’s HZ are exposed to considerable radiation on their sun-facing side, which can also lead to photolysis and an atmosphere dominated by abiotic oxygen. Since oxygen gas is toxic to photosynthetic life forms (such as those that existed in Earth’s early history), it could prevent the emergence of life.
Next up, there’s Ammonia and Phosphine, which are naturally occurring in the atmospheres of gas giants (and icy moons that orbit them) but are also produced by life here on Earth. Phosphine as a potential biosignature has been a hot topic of late, given that planetary scientists detected it not long ago in Venus’ atmosphere. However, both occur in minute quantities here on Earth, making them rather difficult to detect in the atmospheres of distant exoplanets.
Methane and Carbon Dioxide are also considered potential biosignatures (especially in combination, according to Barstow) because of their association with biological processes here on Earth. Both are produced as byproducts by animals, methane resulting from organic decay and digestion, and carbon dioxide as the exhalations of oxygen-consuming animals. The presence of enough CO2 in an atmosphere is also essential to maintaining stable temperatures over time. Too much or too little can lead to a runaway greenhouse effect or glaciation.
Last, according to Barstow, there is the possibility that chemical imbalances could point the way toward life. This is because chemical equilibrium does not exist in a system where life is present since life constantly consumes certain chemicals, producing energy and other chemicals as a byproduct. This echoes what planetary scientists James Lovelock (co-founder of the Gaia Hypothesis) argued in his famous book, the Greening of Mars. “Stability,” he argued, is only present in systems or planets that are lifeless (in this case, he was referring to Mars).
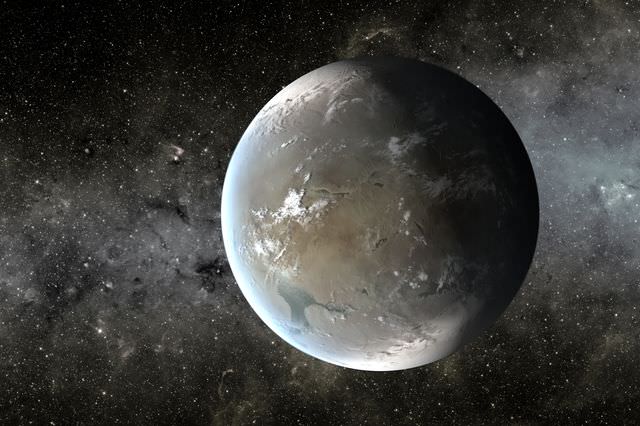
Another possible biosignature that Barstow does not mention is hydrogen. In recent years, researchers from Cornell University showed how the presence of volcanic hydrogen in an exoplanet atmosphere could extend the habitable zones of stars. Not only is hydrogen gas (H2) a natural greenhouse gas, but the presence of volcanic activity is also considered important to the emergence of life. Other research from the University of Cambridge showed how ocean planets with hydrogen-dominated atmospheres (“Hycean” planets) could be the best place to search for life.
However, in all these cases, the presence of these chemicals in an exoplanet’s atmosphere should not be treated as definitive proof that life exists there. As Barstow reminds readers, it is easy to get caught up in the excitement of exoplanet research and forget that the process is long and painstaking. As she summarizes:
“[W]e still have to measure how much of these gases are present to draw meaningful conclusions. This isn’t straightforward as the signals can overlap and need to be carefully disentangled… JWST is only just opening up a new, rich laboratory of planetary atmospheres, and as we explore no doubt we will find many of our previous assumptions are proven wrong.”
Further Reading: The Conversation
The post Here are Four Ways JWST Could Detect Alien Life appeared first on Universe Today.
More Stories
Will Triton finally answer, ‘Are we alone?’
SLS Hurricanes, James Webb Fixed, Strange Quark Star
This Nearby Dwarf Galaxy has Been a Loner for Almost the Entire age of the Universe